In the realm of satellite engineering, the quest for longevity in hardware design is a meticulous dance between precision and durability. Engineers navigate a myriad of challenges, from the relentless forces of launch to the unforgiving environment of space itself. With a strategic selection of materials and components that can withstand the harshest conditions, the goal is clear: to extend the operational lifespan of satellites and minimize the need for interventions in the vacuum of space. But how do engineers truly achieve this delicate balance between innovation and reliability?
Key Takeaways
- Tailored connector selection for different mission phases optimizes hardware efficiency.
- Components resilient to space environment ensure long-term reliability.
- Radiation mitigation strategies like shielding materials protect hardware from radiation effects.
- Hardware redundancy and testing enhance system reliability and operational continuity.
Key Design Considerations for Longevity
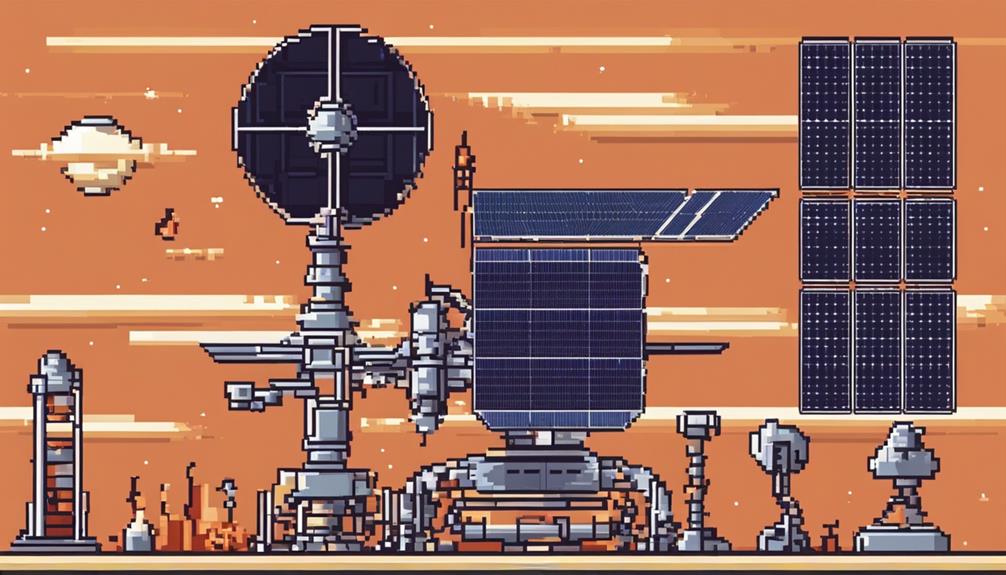
In the realm of satellite hardware design for longevity, meticulous attention to key considerations is paramount to ensuring optimal performance and durability throughout the mission lifespan. Temperature ranges play a critical role in the selection of materials and components for satellite hardware to withstand the extreme conditions of space. Connector specifications must align with these temperature requirements and factor in thermal cycling to prevent malfunctions and ensure the longevity of the satellite hardware.
Secure fixing methods are essential in maintaining the integrity of satellite hardware, especially in high vibration environments commonly experienced during launch and in orbit. Properly securing connectors reduces the risk of disconnection or damage, contributing to the long-lasting performance of the satellite throughout its lifespan. Connector selection tailored to the different phases of a satellite's lifespan is crucial for consistent and reliable performance over time. Adapting connectors to meet the varying demands of a satellite mission ensures that the hardware remains efficient and operational from deployment to the end of its service life.
Implementing space-saving techniques in small satellite designs not only reduces overall weight and cost but also enhances the efficiency and longevity of the hardware. By optimizing space within the satellite structure, components can be arranged strategically to improve performance and durability while minimizing the risk of failures. The integration of space-saving techniques contributes to the reduced wear and tear on satellite hardware, ultimately extending its operational lifespan in orbit.
Components Resilient to Space Environment
Designing satellite hardware components that demonstrate resilience to the harsh space environment is a critical aspect of ensuring mission success and longevity. In space, components are subjected to extreme temperatures, high vibration levels, and mechanical stresses, making durability requirements a top priority. Connectors, in particular, play a vital role in the functionality and reliability of satellite hardware. They must be able to withstand the rigors of launch, including high vibration and mechanical stresses, to ensure proper operation in orbit.
To emphasize the importance of resilience in satellite hardware design, consider the following table showcasing key factors and challenges in ensuring components can endure the space environment:
Factor | Challenges | Solutions |
---|---|---|
Extreme Temperatures | Components must withstand wide temperature ranges | Thermally stable materials and coatings |
High Vibration | Connectors can be damaged by launch vibrations | Secure fixing methods and shock-absorbing materials |
Mechanical Stresses | Components may experience mechanical loads | Robust designs and materials for structural integrity |
Durability Requirements | Long-term reliability is essential | Testing for durability and material selection |
Mitigating Radiation Effects on Hardware
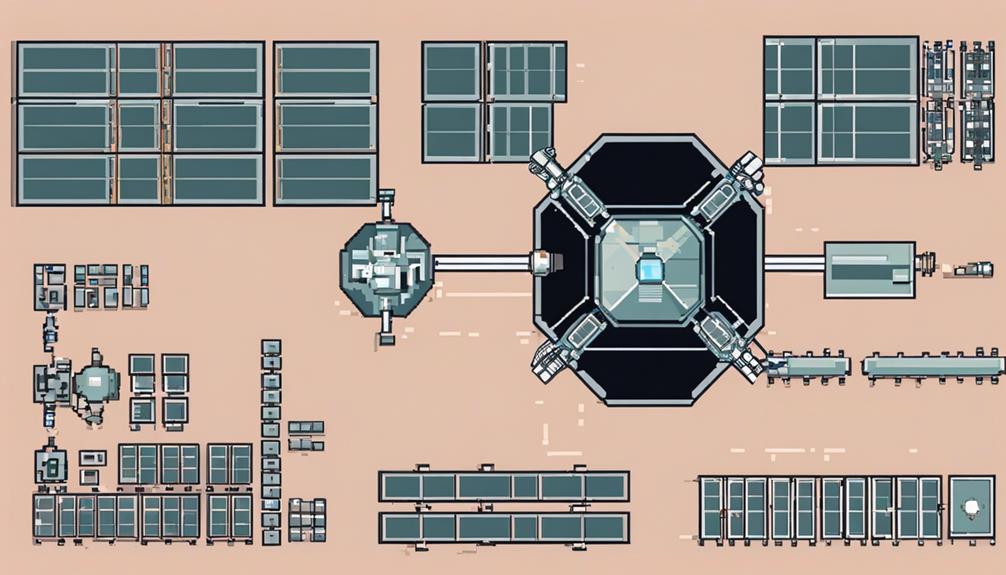
Radiation poses a significant threat to satellite hardware, with single event effects (SEE) and total ionizing dose (TID) being primary concerns. SEE can lead to transient or permanent malfunctions in electronics, while TID gradually degrades semiconductor devices and solar cells. Utilizing radiation shielding materials and employing radiation-hardened components are essential strategies to mitigate these detrimental effects on satellite hardware.
Radiation Shielding Materials
Utilizing advanced materials with superior radiation-absorbing properties is imperative in safeguarding satellite hardware from the detrimental effects of space radiation. When considering radiation shielding materials for satellite hardware, engineers commonly opt for substances such as lead, polyethylene, and tantalum due to their proven efficacy in mitigating the impact of space radiation on electronic components. These materials offer varying levels of protection against cosmic rays, solar radiation, and charged particles prevalent in the space environment. Proper selection and integration of these shielding materials are crucial to prevent data corruption, system malfunctions, and potential satellite failures, ensuring the overall longevity and reliability of satellite hardware in orbit.
- Lead: Known for its high density and excellent radiation-blocking capabilities.
- Polyethylene: Offers lightweight protection with effective radiation absorption properties.
- Tantalum: Provides durable shielding against space radiation for critical electronic components.
- Electronic Components: Vulnerable to damage from space radiation without adequate shielding measures.
Radiation Hardened Components
In safeguarding satellite hardware from the detrimental effects of space radiation, the implementation of radiation-hardened components is crucial to mitigate potential damage and ensure longevity in the harsh space environment. Space radiation can induce single event effects (SEEs) and total ionizing dose (TID) damage to electronic components, emphasizing the need for robust mitigation strategies. These strategies may include shielding, error correction codes, and redundant systems to protect hardware from radiation-induced failures. Radiation testing is essential to verify that components can withstand the challenging space radiation environment. Therefore, spacecraft designers play a critical role in carefully selecting and qualifying radiation-hardened components to guarantee mission success amidst the unforgiving conditions of outer space.
Ensuring Thermal Stability of Satellite
Maintaining thermal stability within satellites is a critical aspect of hardware design to ensure operational efficiency in the rigorous conditions of space. To achieve this goal, several key measures are implemented:
- Thermal Stability: Satellite hardware is engineered to withstand extreme temperatures, from the frigid cold of deep space to the searing heat when exposed to direct sunlight. This capability ensures that critical components continue to function optimally regardless of external conditions.
- Thermal Management Systems: Sophisticated thermal management systems are integrated into satellites to regulate internal temperatures effectively. These systems employ mechanisms such as heaters, radiators, and insulation to control heat dissipation and maintain a stable thermal environment within the satellite.
- Materials Selection: The choice of materials is crucial in maintaining thermal stability. Components with high thermal conductivity help in efficient heat transfer, while insulation materials prevent temperature fluctuations. By using a combination of these materials judiciously, satellite designers can ensure consistent thermal conditions.
- Mission Success: Ultimately, the successful implementation of thermal stability measures is paramount for mission success. A satellite that can maintain its operational capabilities despite temperature differentials and extreme conditions is more likely to fulfill its objectives over an extended period, contributing to the longevity and effectiveness of the mission.
Strategies for Launch Stress Management
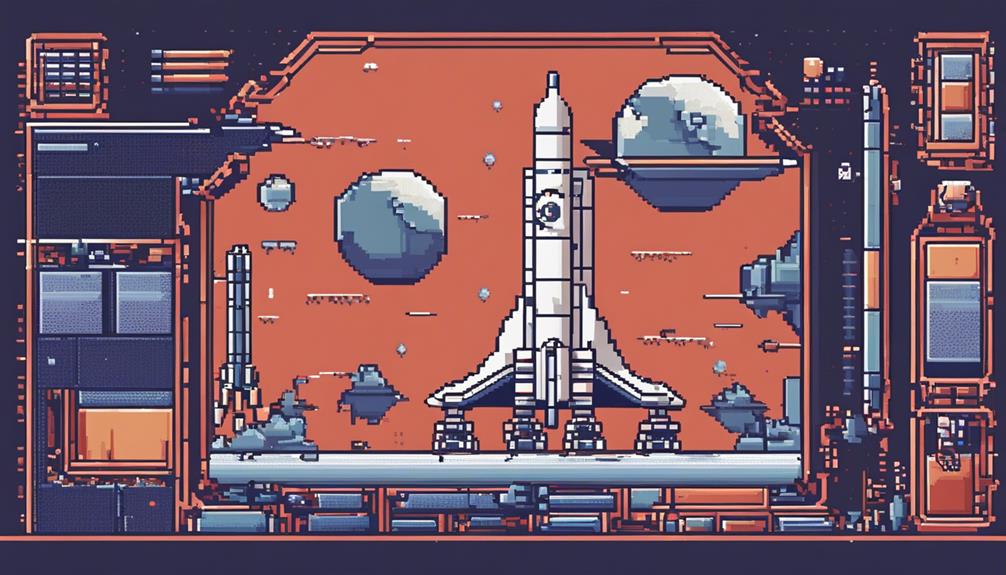
Implementing robust launch stress management strategies is crucial for safeguarding the durability and functionality of satellite hardware in the demanding space environment. To ensure the integrity of satellite hardware during launch, connectors play a vital role. These connectors must be designed to withstand significant mechanical stresses such as shock, vibration, and high G forces. Special attention needs to be given to outgassing considerations to prevent contamination and equipment damage during the launch phase. It is essential to employ secure fixing methods for connectors to maintain stable connections, especially in high vibration environments.
Temperature extremes in space pose another challenge that launch stress management strategies must address. Connectors need to be meticulously designed and selected to ensure optimal performance and reliability in varying temperature conditions. The connectors must be able to withstand both extreme cold and heat without compromising their functionality. By carefully considering these factors and implementing appropriate strategies, satellite hardware can be better equipped to withstand the rigors of the launch process, ultimately increasing the longevity and effectiveness of the satellite system in space.
Importance of Redundancy in Hardware
To ensure the resilience and operational continuity of satellite systems in space missions, the incorporation of redundancy in critical hardware components is imperative. Redundancy in satellite hardware involves duplicating essential components to mitigate the impact of potential failures and increase overall system reliability. Here are four key points highlighting the importance of redundancy in satellite hardware:
- Mission Success: Redundancy strategies play a crucial role in ensuring the success of space missions by providing backup systems and components that can take over in case of primary system failures.
- System Reliability: Implementing redundancy in satellite hardware enhances the system's reliability by reducing the likelihood of critical component failures disrupting mission operations.
- Operational Continuity: Redundant hardware systems help maintain operational continuity in space missions by allowing the satellite to continue functioning even if certain components malfunction.
- Extend Lifespan: Redundancy design in satellite hardware aims to minimize mission risks and extend the operational lifespan of satellites by providing fallback mechanisms for critical functions. By incorporating redundancy, satellite engineers can effectively mitigate the impact of failures and improve the overall robustness of the satellite system.
Testing and Validation Procedures
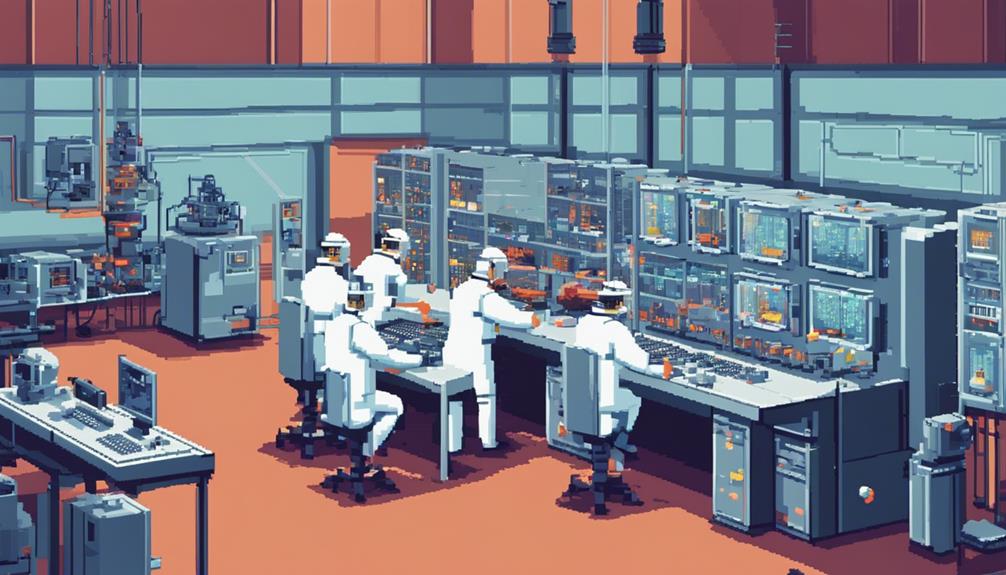
Testing and validation procedures in satellite hardware design encompass stress testing procedures, environmental simulation tests, and reliability analysis methods. Stress testing involves subjecting hardware to extreme conditions to assess its resilience, while environmental simulation tests replicate the rigors of space environments. Reliability analysis methods are employed to ensure that satellite hardware can withstand the demands of launch and operate effectively in space.
Stress Testing Procedures
The rigorous stress testing procedures applied to satellite hardware are essential in ensuring its resilience in the demanding conditions of launch and space environments. These procedures involve subjecting the hardware to extreme conditions like vibration, shock, and high G forces to assess durability and hardware resilience. To convey a deeper understanding, consider the following:
- Vibration testing ensures the hardware can withstand the mechanical stresses experienced during launch.
- Shock testing validates the hardware's ability to endure sudden impacts without damage.
- High G force testing simulates the acceleration forces encountered in space operations.
- Durability assessments verify the long-term performance of the hardware under extreme conditions.
Environmental Simulation Tests
Environmental simulation tests play a crucial role in evaluating the durability and performance of satellite hardware by subjecting it to extreme conditions like temperature, vibration, and vacuum. These tests are essential for ensuring the hardware's reliability in the harsh space environment. Thermal vacuum chambers are utilized to replicate temperature extremes ranging from -200°C to 200°C, assessing how satellite components respond to drastic thermal variations. Vibration tests are conducted to simulate the mechanical stresses experienced during launch and operation, verifying the resilience of connectors, fasteners, and structures. By subjecting satellite hardware to these environmental testing procedures, engineers can validate the design and materials, ensuring they meet the stringent requirements for longevity and functionality in space missions.
Reliability Analysis Methods
To ensure the longevity and reliability of satellite hardware, in-depth reliability analysis methods encompass rigorous testing and validation procedures.
- Comprehensive Testing: Includes thermal cycling, vibration, shock, and outgassing evaluations to simulate harsh space conditions.
- Validation Procedures: Verify connector durability, material integrity, and performance under extreme temperature ranges.
- Identification of Failure Points: Reliability testing helps pinpoint potential weaknesses and failure points within the hardware.
- Enhanced Hardware Resilience: Thorough analysis methods are crucial for ensuring the durability and resilience of satellite hardware components over their operational lifespan.
Frequently Asked Questions
What Determines the Life of a Satellite?
Environmental factors, component reliability, radiation exposure, thermal management, mission profile, solar panel degradation, and on-board propulsion are key determinants of a satellite's lifespan. The harsh space environment, durability of space-grade hardware, and ability to withstand mechanical stresses and extreme temperatures all impact longevity. Selecting robust materials and reliable connectors is crucial in ensuring satellites can endure the rigors of space, ultimately extending their operational life.
What Is the Life Expectancy of a Satellite?
The life expectancy of a satellite is influenced by several factors such as mission requirements, solar panel efficiency, radiation effects on components, propulsion system performance, thruster degradation over time, and battery lifespan. Understanding these aspects is crucial in designing satellite hardware that can withstand the harsh space environment and meet longevity goals. Careful consideration of these factors ensures that satellites can operate effectively for the desired duration of their missions.
What Is the Design Lifetime of a Satellite?
The design lifetime of a satellite, contingent upon the mission requirements and utilized components, generally spans from 5 to 15 years. Component reliability, solar panels, radiation shielding, thermal management, power systems, communication systems, and attitude control are critical elements in ensuring the longevity of a satellite in orbit. Robust design choices and meticulous engineering considerations are imperative for sustaining operational efficiency and performance over an extended period in space.
What Is the Longest Lasting Satellite?
The longest-lasting satellite currently in operation is the Voyager 2 spacecraft, which has been active for over 40 years. Its durability can be attributed to meticulous design considerations that account for the harsh space environment, radiation effects, appropriate material selection, high component reliability, effective thermal control systems, reliable power generation mechanisms, and a well-planned mission duration. These factors collectively contribute to the exceptional longevity of Voyager 2 in space exploration.