In the intricate realm of satellite network design, the interplay of orbit selection, frequency allocation, and network topology crafts the foundation for operational success. Orbit choice, whether in GEO, MEO, or LEO realms, significantly impacts coverage and latency. Frequency assignment intricacies directly influence signal clarity and interference management. Deliberate network topology decisions sculpt the pathway for efficient data flow. The meticulous orchestration of these elements is akin to composing a symphony of connectivity, where precision and foresight pave the way for a harmonious satellite network ecosystem.
Key Takeaways
- Strategic ground station placement ensures uninterrupted communication and network reliability.
- Antenna technology selection impacts signal transmission efficiency and coverage.
- Network optimization involves capacity planning, frequency management, and power system alignment.
- Implementing security measures and network redundancy enhances system resilience and data integrity.
Capacity Planning
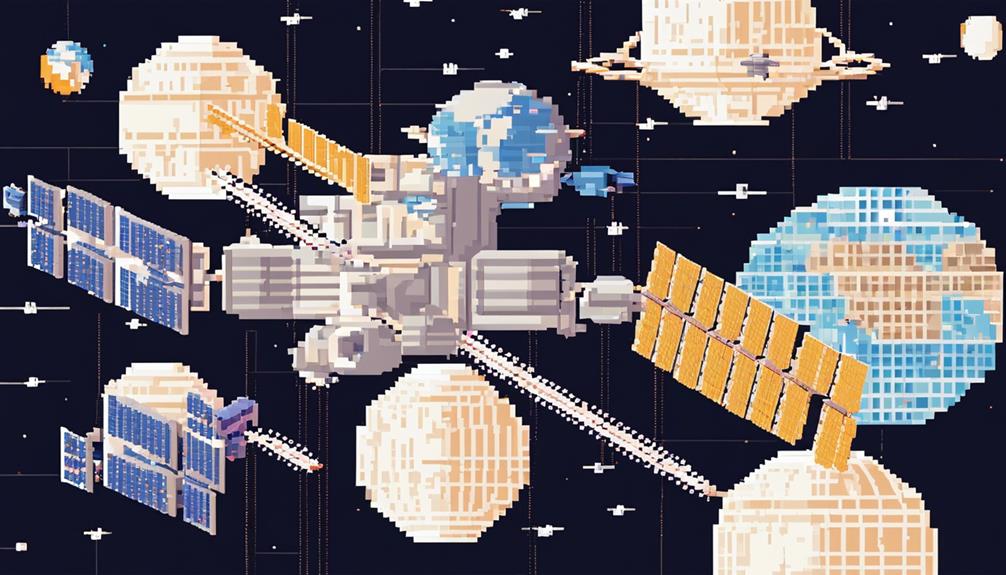
Capacity planning in satellite networks involves analyzing and determining the requisite bandwidth for efficient data transmission. When considering the power system within this context, it is crucial to ensure that the allocated bandwidth aligns with the power requirements of the satellite network. The power system of a satellite network is a fundamental aspect of its design, as it directly impacts the network's ability to transmit and receive data effectively.
In capacity planning, the power system must be carefully evaluated to support the required bandwidth without compromising the network's overall performance. Factors such as the power efficiency of the satellite's components, the power consumption of the communication systems, and the availability of power from solar panels or onboard batteries play a significant role in determining the optimal capacity allocation for data transmission.
Efficient capacity planning in satellite networks involves not only meeting the current power demands but also anticipating future power requirements to support network growth and expansion. By balancing the capacity allocation with the power system capabilities, satellite operators can ensure that the network operates at peak efficiency while accommodating potential increases in data transmission needs. Incorporating advanced power management techniques and technologies into the capacity planning process can further enhance the network's performance and reliability in delivering data services to users.
Orbital Configurations
The selection of orbital configurations plays a critical role in determining the performance and capabilities of a satellite network design. Different Earth orbits offer various advantages and disadvantages that need to be carefully considered when designing a satellite network. Here are three key points to understand about orbital configurations in satellite communication systems:
- Geostationary Earth Orbit (GEO): GEO satellites orbit at an altitude of approximately 35,786 kilometers above Earth's equator. While GEO orbits provide high coverage over a specific area, they suffer from high latency due to the long distance from Earth. This makes GEO orbits suitable for applications where consistent coverage is more critical than low latency, such as broadcasting.
- Medium Earth Orbit (MEO): MEO satellites operate at altitudes ranging from 2,000 to 35,786 kilometers above the Earth's surface. MEO orbits offer a balance between coverage and latency, making them suitable for applications that require a moderate level of coverage and acceptable latency, such as navigation systems like GPS.
- Low Earth Orbit (LEO): LEO satellites orbit at altitudes below 2,000 kilometers. LEO orbits provide low latency due to their proximity to Earth but require a larger number of satellites to achieve global coverage. This makes LEO orbits ideal for applications that demand low latency, such as real-time communication and Earth observation.
Ground Station Placement
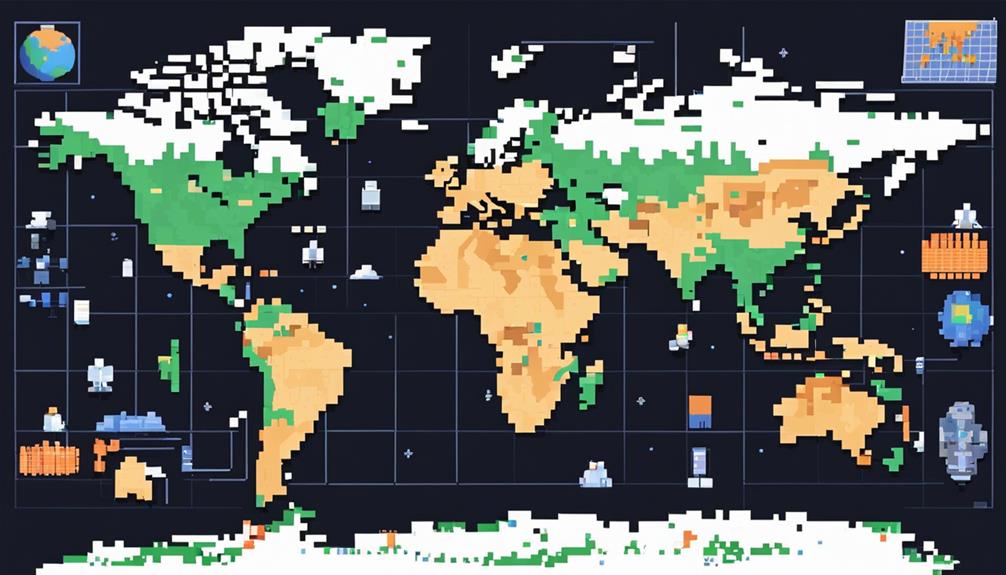
Strategic placement of ground stations is essential to ensure uninterrupted communication coverage with satellites in a satellite network. The optimal positioning of ground stations plays a crucial role in maintaining a seamless flow of data between the satellites and the Earth. Factors such as the visibility of satellites, signal strength, and data transfer rates are meticulously considered when determining the locations for ground stations.
To guarantee global coverage and connectivity as satellites orbit the Earth, ground stations are strategically distributed across different geographical regions. This distribution helps in overcoming challenges such as signal blockages and ensures continuous communication with the satellites in orbit. Additionally, redundant ground stations may be established to mitigate potential signal disruptions caused by adverse weather conditions or technical issues at primary stations.
The efficiency, reliability, and overall performance of the satellite network are significantly influenced by the placement of ground stations. By strategically locating these stations, network operators can optimize data transmission, minimize signal interference, and enhance the network's resilience to various operational challenges. Therefore, meticulous planning and precise placement of ground stations are fundamental aspects of designing a robust and efficient satellite network.
Antenna Selection
In the context of optimizing satellite network performance and ensuring seamless communication with satellites, the selection of antennas is a critical component that demands meticulous consideration. Antennas serve as the interface between ground stations and satellites, playing a pivotal role in transmitting and receiving signals effectively. When choosing antennas for a satellite network, several factors need to be taken into account, including the frequency bands used for communication. Here are three key aspects to consider when selecting antennas for satellite communication:
- Frequency Bands: Different antennas are designed to operate within specific frequency ranges. It is essential to match the antenna's frequency capabilities with the satellite network's communication requirements. Understanding the frequency bands allocated for satellite communication is crucial in selecting antennas that can efficiently transmit and receive signals within these designated ranges.
- Gain and Beamwidth: Antennas with higher gain can transmit signals over longer distances with more focused power. The beamwidth of an antenna determines the coverage area of the signal. For satellite networks, antennas with suitable gain and beamwidth characteristics must be chosen to ensure reliable communication links with satellites orbiting at varying distances.
- Polarization Compatibility: Polarization alignment between antennas on the ground and satellites in space is essential for signal reception. Matching the polarization of antennas to that of the satellite network is crucial for maximizing signal strength and minimizing interference. Antennas with adjustable polarization settings can enhance communication reliability in satellite networks operating across different frequency bands.
Frequency Management
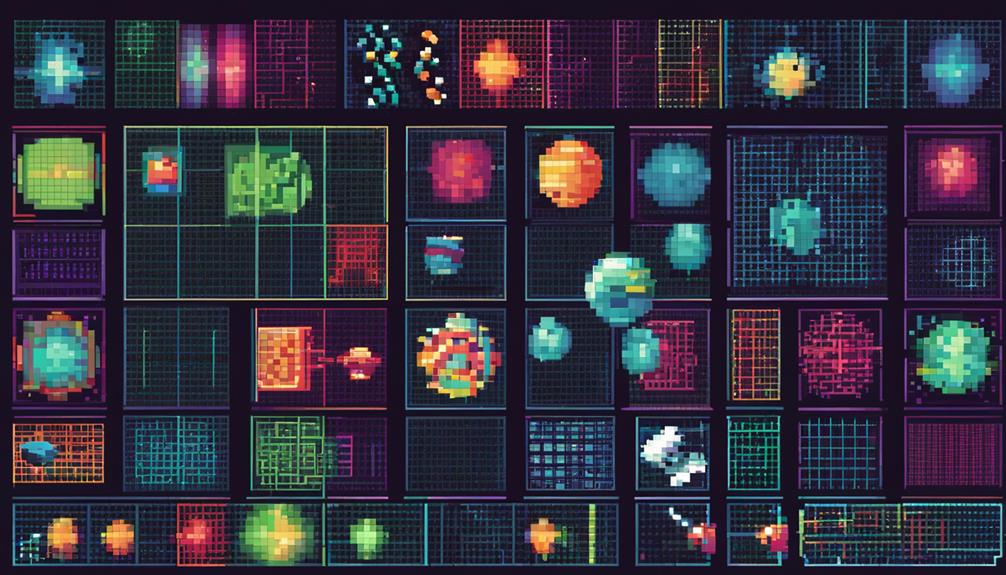
Effectively managing frequencies in satellite networks is paramount for optimizing performance and ensuring seamless communication across designated bands. Frequency management in satellite networks involves allocating specific bands such as C-band, Ku-band, and Ka-band for communication purposes. Each frequency band possesses unique characteristics that impact factors like bandwidth, coverage, and interference within satellite communication systems. The choice of frequency band significantly influences data transmission speed, signal quality, and overall network performance.
Different frequency bands offer trade-offs concerning availability, capacity, and susceptibility to weather conditions for satellite communications. For instance, C-band is known for its reliability in adverse weather conditions, while Ku-band offers higher data transmission speeds but is more susceptible to rain fade. Ka-band provides even greater data rates but is more sensitive to atmospheric conditions. Effective frequency management is crucial for optimizing satellite network performance and ensuring efficient data transmission across various orbits and mission requirements.
Interference Mitigation
Interference mitigation strategies in satellite networks encompass signal filtering techniques and efficient frequency band allocation to combat signal degradation and enhance overall system performance. By employing adaptive power control mechanisms and frequency reuse schemes, satellite communication systems can effectively manage interference issues and optimize spectral efficiency. Additionally, spatial diversity techniques and advanced modulation schemes play crucial roles in improving signal reception and ensuring reliable data transmission in the presence of interference.
Signal Filtering Techniques
Signal filtering plays a crucial role in enhancing the quality and performance of satellite communication systems by effectively reducing unwanted signals. Common filtering methods, such as analog and digital filters, are employed to remove interference. Analog filters work on continuous signals, while digital filters process discrete signals. Here are three key points on signal filtering techniques in satellite networks:
- Improved Signal Quality: Filtering helps improve the quality of signals transmitted and received, leading to clearer communication.
- Noise Reduction: By filtering out unwanted signals and noise, the overall signal-to-noise ratio is enhanced, improving the system's performance.
- Adaptive Filtering: Adaptive filtering algorithms can dynamically adjust filter parameters based on changing interference conditions, ensuring optimal signal reception.
Frequency Band Allocation
Frequency band allocation is a critical aspect of managing interference in satellite networks. The allocation of different bands such as C-band, Ku-band, Ka-band, and Q/V-band plays a vital role in determining the characteristics of communication within the network. Decisions regarding frequency band allocation directly impact factors such as bandwidth availability, signal quality, and overall network performance. Operators are tasked with the challenge of balancing various trade-offs, considering factors like band capacity, propagation characteristics, and interference levels. Proper frequency band allocation is essential for optimizing the efficiency and reliability of satellite networks, ensuring seamless communication while mitigating potential interference issues that could disrupt network operations. By strategically allocating frequency bands, operators can enhance the overall performance and effectiveness of satellite communication systems.
Satellite Constellation Design
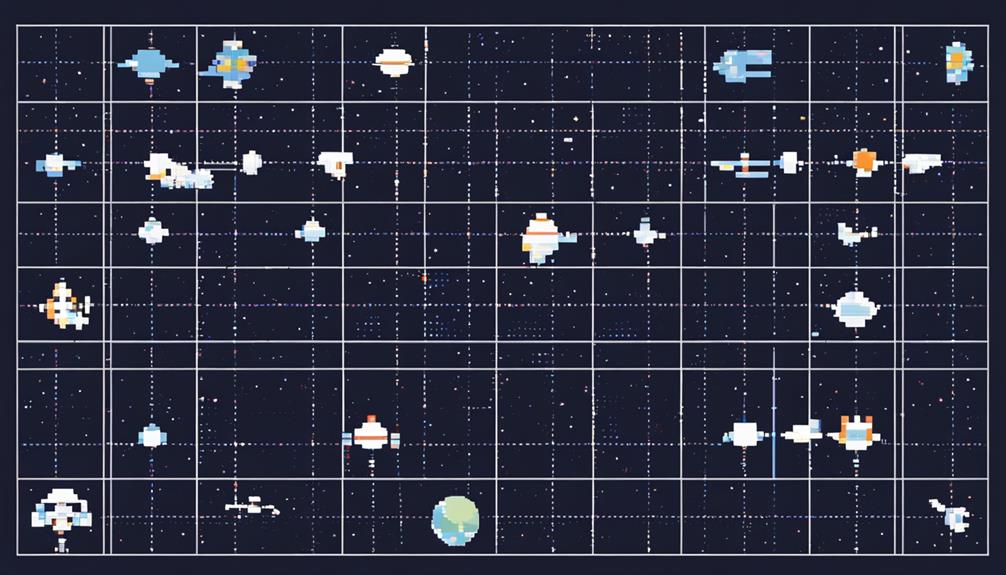
Satellite constellation design involves intricate considerations such as orbital spacing and ground coverage optimization. The strategic arrangement of satellites in a constellation directly impacts the network's performance and efficiency. These factors are crucial in ensuring seamless communication and data relay capabilities within the satellite network.
Orbital Spacing Considerations
In the meticulous planning of satellite constellation deployment, the consideration of optimal orbital spacing stands as a foundational element for ensuring comprehensive coverage and reliable network connectivity. Proper orbital spacing is crucial for the efficient operation of the satellite network, impacting various aspects such as network performance, interference mitigation, and seamless handoffs between satellites. When determining orbital spacing, factors like orbital altitude, inclination, and phasing play significant roles in achieving an effective satellite constellation design. To optimize network functionality and minimize potential issues, satellite engineers must carefully calculate and position satellites to ensure adequate coverage area and continual connectivity.
Key Orbital Spacing Considerations:
- Impact on Network Performance
- Interference Mitigation Strategies
- Seamless Satellite Handoffs
Ground Coverage Optimization
Optimizing ground coverage within a satellite constellation design necessitates strategic satellite positioning to maximize coverage efficacy across targeted regions, influenced by orbital parameters, inclination, altitude, and constellation geometry. Satellite communications rely heavily on efficient ground coverage optimization to ensure seamless connectivity and reliable network performance. By carefully configuring orbital spacing and constellation layout, satellite networks like Iridium and Starlink achieve global coverage. Advanced algorithms and simulations are employed to analyze and refine designs, enhancing the effectiveness of satellite constellations for communication purposes. Ground coverage optimization is a critical component in meeting the increasing demands for connectivity, enabling continuous communication services, and bolstering network reliability.
Factors | Impact on Ground Coverage Optimization |
---|---|
Orbital Parameters | Influence satellite visibility and reach |
Inclination | Affects coverage over specific latitudes |
Altitude | Dictates signal strength and coverage area |
Constellation Geometry | Determines overall coverage efficiency |
Network Reliability | Enhanced by optimized ground coverage |
Signal Propagation Analysis
Signal propagation analysis is a critical aspect of designing a satellite network, as it delves into the intricate behavior of electromagnetic signals as they traverse various mediums. This analysis is fundamental for optimizing communication links and ensuring reliable connectivity within the satellite network. Here are three key aspects related to signal propagation analysis:
- Understanding Signal Behavior: Engineers study how electromagnetic signals propagate through mediums like the atmosphere, space, and different terrain types. Factors such as signal absorption, reflection, refraction, and diffraction play a crucial role in determining the quality and strength of the signal received at the destination.
- Impact of Environmental Variables: Atmospheric conditions, such as rain, snow, or fog, can attenuate or scatter signals, affecting the overall communication quality. Additionally, the terrain through which the signal travels can introduce obstacles or signal blockages that need to be accounted for during network design.
- Utilization of Tools for Analysis: Engineers utilize tools like link budget calculations to estimate signal strength, path loss, and received signal quality. By conducting thorough signal propagation analysis, network designers can optimize antenna placement, adjust power levels, and allocate frequencies effectively to enhance communication efficiency and reliability in satellite networks.
Security Measures
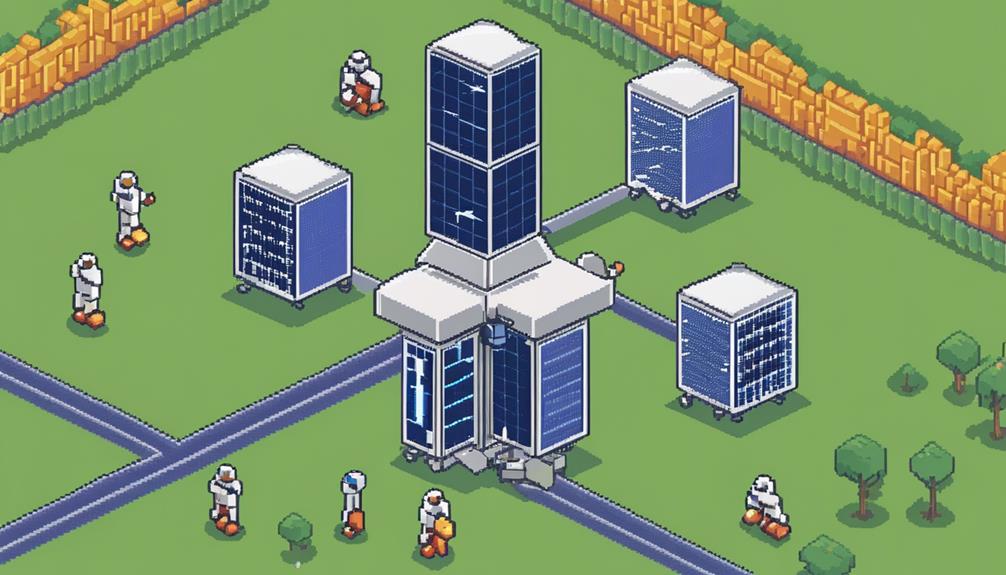
Security measures in a satellite network encompass the implementation of robust encryption protocols like AES to safeguard communications, alongside access control methods such as authentication mechanisms including digital signatures and certificates. Additionally, threat detection systems play a crucial role in monitoring network traffic for any signs of unauthorized access or suspicious activities. Together, these measures contribute to the overall security posture of the satellite network, ensuring the protection of sensitive data and the integrity of communications.
Encryption Protocols Used
Encryption protocols such as AES and RSA play a crucial role in safeguarding satellite network communications against unauthorized access and data breaches. When designing a satellite network, the choice of encryption protocols is vital to ensure the confidentiality and integrity of data transmitted over low earth orbit satellites. To effectively secure satellite communications, the following encryption measures are commonly implemented:
- AES Encryption: Utilized for its efficiency and robust security features in protecting data transmitted over satellite links.
- RSA Encryption: Used for key exchange and digital signatures to authenticate and secure satellite network connections.
- SSL and TLS Protocols: Implemented to establish secure connections between satellite terminals and ground stations, enhancing overall communication security.
Access Control Methods
Access control methods in satellite networks encompass a range of protocols aimed at ensuring the secure transmission of data and protecting network integrity. Encryption techniques such as AES, RSA, and ECC play a vital role in safeguarding sensitive information, while authentication methods like passwords, biometrics, and digital certificates verify user and device identities. Authorization mechanisms define access rights based on roles and permissions. Implementing robust access control measures is crucial for fortifying satellite network infrastructure against cyber threats and ensuring data integrity. By combining encryption, authentication, and authorization effectively, satellite networks can mitigate unauthorized access and maintain the confidentiality and integrity of transmitted data.
Access Control Method | Description |
---|---|
Encryption | Protects data through cryptographic algorithms such as AES, RSA, and ECC. |
Authentication | Verifies user and device identities using passwords, biometrics, and digital certificates. |
Authorization | Defines access rights based on user roles and permissions within the network. |
Threat Detection Systems
Utilizing sophisticated algorithms and real-time monitoring capabilities, threat detection systems in satellite networks are designed to swiftly identify and neutralize potential security breaches. These systems continuously monitor network traffic, analyzing patterns and behaviors to detect anomalies that may indicate a security threat. To enhance their effectiveness, threat detection systems integrate machine learning and artificial intelligence, enabling them to proactively defend against evolving cyber attacks in real-time. In satellite network design, implementing robust security measures like encryption protocols, access controls, and intrusion detection systems is crucial to safeguard critical data and infrastructure.
3 Key Components of Threat Detection Systems:
- Advanced algorithms for rapid threat identification.
- Real-time monitoring of network traffic for anomalies.
- Integration of machine learning and artificial intelligence for proactive defense.
Network Redundancy
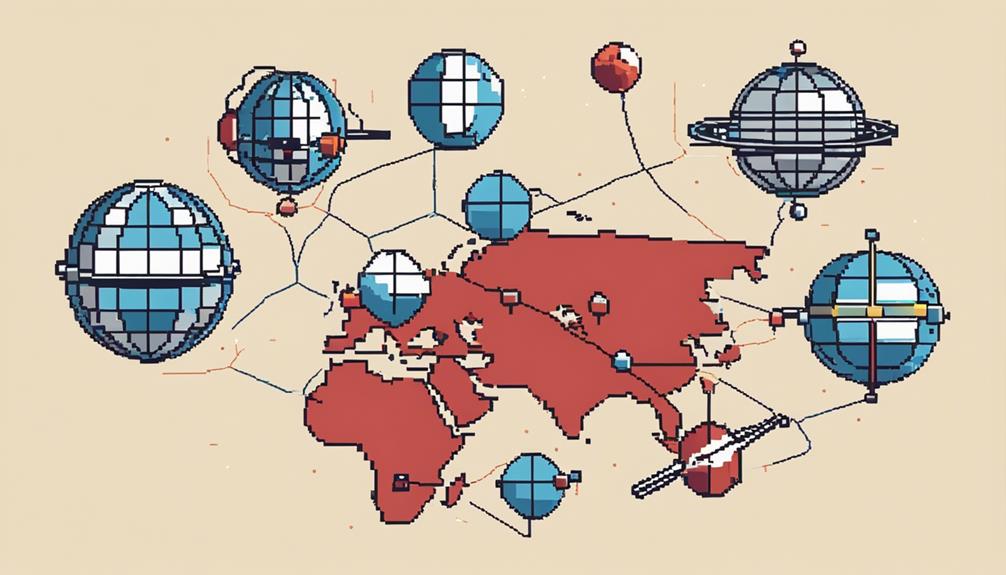
To ensure continuous operation and mitigate the risks of system failures, network redundancy in satellite systems involves the incorporation of duplicate components or paths. Redundancy in satellite networks is a crucial aspect of the overall design to enhance system resilience. This redundancy can be achieved through various means, such as backup satellites, redundant ground stations, or additional communication links. By having these duplicate elements in place, the network can continue functioning even if one or more components fail, ensuring uninterrupted service and data transmission.
The design of redundancy strategies is a meticulous process that considers factors like mission criticality, cost implications, and the desired level of fault tolerance. Different satellite missions may require varying levels of redundancy based on the importance of the data being transmitted and the potential impact of a system failure. Implementing redundancy not only increases system reliability but also minimizes downtime, which is crucial for satellite operations where constant connectivity is essential.
Data Transfer Protocols
Efficient data transfer protocols play a crucial role in ensuring seamless and reliable communication between satellites and ground stations in satellite networks. When designing satellite networks, the choice of data transfer protocols significantly impacts the overall performance and effectiveness of the system. Here are three key aspects related to data transfer protocols in satellite networks:
- Management of Data Packet Flow: Data transfer protocols in satellite networks are responsible for managing the flow of data packets between satellites and ground stations. These protocols consider various factors such as latency, bandwidth availability, and congestion in space communications to optimize the data transfer process.
- Variety of Protocols: Satellite data transfer protocols commonly used include TCP/IP, UDP, and custom protocols specifically optimized for satellite network characteristics. Each protocol has its strengths and weaknesses, and the selection depends on the specific requirements of the satellite network design and the nature of the data being transferred.
- Enhanced Connectivity and Performance: Implementing robust data transfer protocols is essential for maintaining connectivity, minimizing delays, and optimizing resource utilization in satellite networks. By ensuring efficient data transfer, satellite communication systems can achieve enhanced overall performance and effectiveness, meeting the demands of modern satellite network designs.
System Performance Optimization
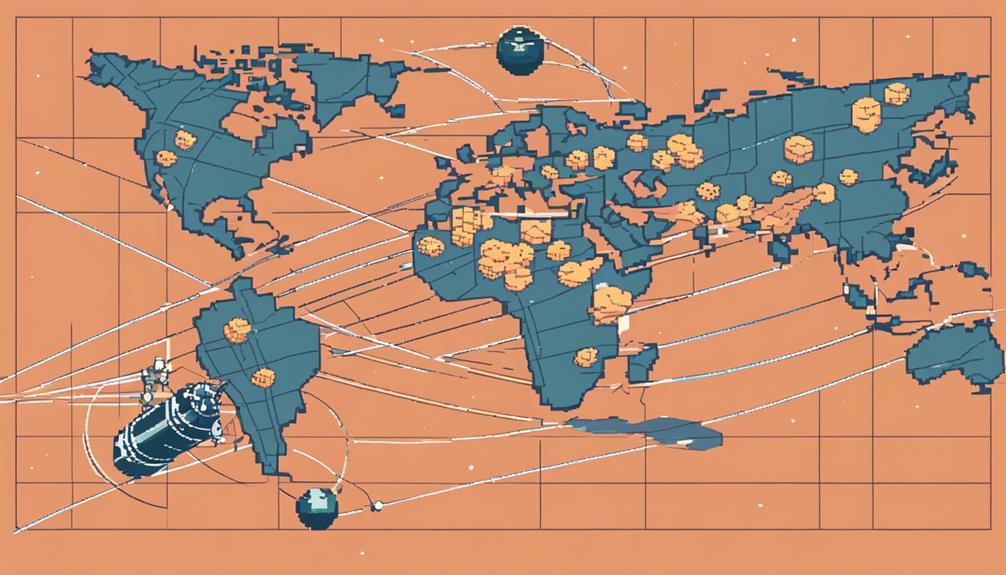
In the realm of satellite networks, optimizing system performance is a critical endeavor aimed at enhancing data transmission efficiency and reliability. Various techniques and technologies are employed to achieve this optimization, considering factors such as link budget, interference, and coverage to enhance overall system performance. One key aspect of system performance optimization is the utilization of adaptive coding and modulation techniques. These methods allow for the adjustment of transmission parameters based on varying link conditions, ensuring that data is efficiently transmitted and received.
Dynamic resource allocation plays a crucial role in optimizing satellite network performance by effectively managing bandwidth usage and minimizing latency. By dynamically allocating resources based on demand and network conditions, satellite networks can operate more efficiently and provide better service to users on Earth. Additionally, advanced antenna technologies like phased arrays contribute significantly to optimizing signal strength and coverage in satellite networks. These antennas can dynamically adjust their beam patterns to focus signals where they are needed most, improving overall network performance and ensuring reliable communication between satellites and Earth stations. By implementing these optimization strategies, satellite networks can achieve higher levels of efficiency and reliability in data transmission, ultimately enhancing their operational capabilities.
Frequently Asked Questions
What Four Factors Should Be Considered When Designing a Satellite System?
When considering the architecture of a satellite system, key factors to evaluate include altitude selection, frequency allocation choices, link budget calculations, and network topology decisions. These elements play crucial roles in determining coverage, latency, bandwidth, signal strength, noise, interference, traffic routing, and processing functions within the system. Each factor must be carefully assessed to ensure optimal performance and efficient operation of the satellite network.
How to Design a Satellite?
When designing a satellite, one must first consider the propulsion system. Satellite propulsion is crucial for achieving and maintaining the desired orbit. Various propulsion methods such as chemical, electric, or solar sails can be utilized based on mission requirements. The propulsion system plays a vital role in maneuvering the satellite, adjusting its position, and ensuring proper functionality throughout its operational lifespan.
What Are the 3 Types of Satellite Communication?
The three main types of satellite communication are Geostationary Earth Orbit (GEO), Medium Earth Orbit (MEO), and Low Earth Orbit (LEO). Each type offers distinct advantages and disadvantages in the realm of satellite technology. GEO satellites provide continuous coverage due to their fixed point above the Earth's equator but have higher latency. MEO satellites offer a balance between coverage and latency, while LEO satellites enable low latency communication at the cost of requiring a larger constellation for global coverage.
What Are the Critical Considerations in Satellite Design?
In satellite design, critical considerations revolve around optimizing satellite orbits to meet specific mission objectives efficiently. Factors such as orbit selection, frequency allocation, and link budget are crucial in determining the satellite's coverage, power requirements, and overall performance. These decisions heavily influence the network topology, routing protocols, and equipment specifications of the satellite system. Ultimately, meticulous planning and optimization of satellite orbits play a hyperbolic role in ensuring the success and reliability of satellite missions.