The backbone of satellite networks, the space segment, serves as the fundamental link between ground communications and the vast expanse of space. A complex interplay of advanced technologies and precise engineering, the space segment encapsulates the intricacies of satellite operations, from orbital mechanics to payload delivery. Understanding the dynamics of this segment is crucial for grasping the seamless connectivity that underpins modern telecommunications. As we explore the depths of this space-bound realm, a world of innovation and possibility awaits, promising a deeper insight into the workings of our interconnected world.
Key Takeaways
- Satellite segment crucial for global connectivity and observational functions.
- Maneuvering techniques optimize satellite operations and network efficiency.
- Various satellite orbits and payloads cater to specific communication needs.
- Operations focus on solar panel deployment, launches, payload integration, and monitoring for mission success.
Overview of Space Segment
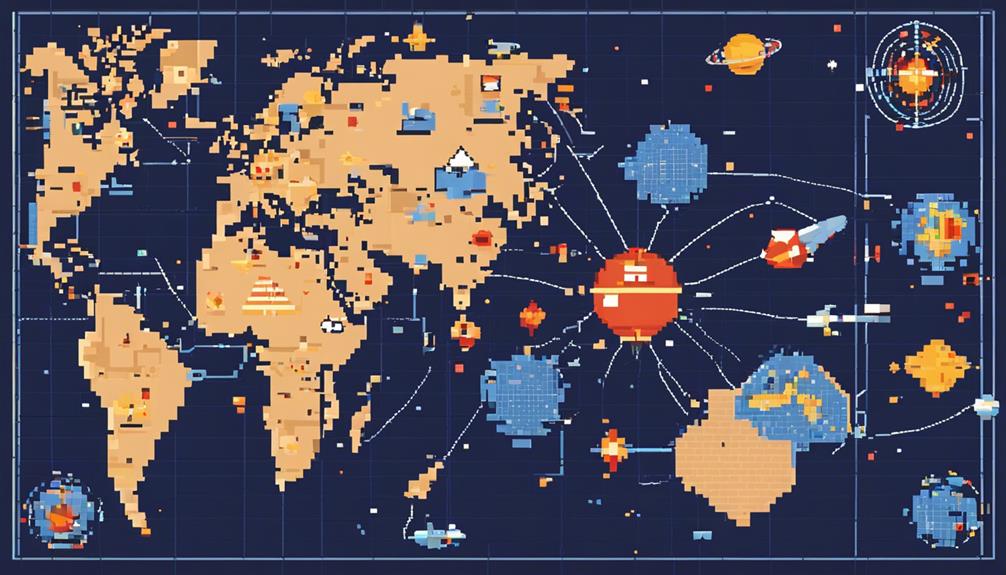
The space segment in satellite networks, essential for the operation and functionality of satellite systems, encompasses individual spacecraft or constellations that serve various communication and observational purposes. Within the Space Segment, communications satellites play a vital role in facilitating telecommunications globally. These satellites are equipped with payloads designed to receive signals from Earth, amplify them, and retransmit them to different locations, enabling long-distance communication.
Satellite constellations, a collection of multiple satellites working together, offer enhanced coverage and connectivity compared to single satellites. By strategically positioning satellites in constellations, network operators can ensure seamless communication services worldwide. These constellations can be utilized for a range of applications, including internet services, weather monitoring, and navigation systems.
The Space Segment is not limited to individual satellites but also includes satellite buses. These buses provide the necessary structure and subsystems for satellite operation, such as power, communications, thermal control, and attitude control. By incorporating these subsystems into the design of each satellite, operators can optimize performance and ensure the longevity of the spacecraft in orbit.
Types of Telecommunications Satellites
Telecommunications satellites vary in their orbits, payload types, and frequency bands, each designed to cater to specific communication needs. Understanding these distinctions is crucial for optimizing satellite network performance and efficiency. The unique characteristics of each type of satellite play a significant role in shaping the telecommunications landscape.
Satellite Orbits
Positioned at varying altitudes, different types of satellite orbits play crucial roles in telecommunications networks, serving distinct purposes based on their unique characteristics and coverage capabilities. Geostationary Earth Orbit (GEO) satellites, located at 35,786 km above the equator, provide fixed coverage for telecommunications. Medium Earth Orbit (MEO) satellites, at altitudes of 10,000-20,000 km, enhance coverage for mobile communications. Low Earth Orbit (LEO) satellites, below 2,000 km, enable low-latency communication, such as satellite internet. Highly elliptical orbits (HEO) serve specific purposes like high-latitude coverage, while Molniya orbits are eccentric orbits used for extended visibility over specific regions. Each orbit type offers distinct advantages in supporting various telecommunications needs.
Payload Types
In satellite networks, the different types of telecommunications satellites are distinguished by their specific payload types, which play a vital role in facilitating communication services. These payload types include:
- Transponders: Components responsible for receiving, amplifying, and retransmitting signals for communication purposes.
- Antennas: Devices used for transmitting and receiving signals to and from the ground stations.
- Multiple Payloads: Some satellites may have multiple payloads to cater to various customers or offer different services simultaneously.
The payload on a satellite is crucial for enabling functions like broadcasting and data transmission. Additionally, it ensures that communication services are effectively provided while also managing aspects such as thermal control within the satellite system.
Frequency Bands
Among the various types of telecommunications satellites, distinctions arise based on the frequency bands in which they operate, encompassing C, Ku, Ka, and L bands. Each frequency band offers unique advantages tailored to specific communication needs. The table below summarizes the key characteristics of each frequency band in satellite communications:
Frequency Band | Characteristics |
---|---|
C-band | Reliable coverage, potential interference |
Ku-band | Higher data rates, smaller antennas |
Ka-band | High-speed data services, broadband applications |
L-band | Suitable for mobile communications, obstacle penetration |
Understanding the capabilities of each frequency band is crucial when selecting the appropriate satellite for different communication requirements. Additionally, considerations such as coverage area, data rates, and antenna sizes play a significant role in determining the most suitable frequency band for satellite communications.
Satellite Orbits and Coverage
The arrangement of satellites in various orbital planes plays a crucial role in ensuring comprehensive global coverage for satellite networks like the GPS constellation. This strategic placement enables optimal Earth coverage and accuracy, as evidenced by the following key points:
- Multiple Orbital Planes: The GPS constellation consists of 24 operational satellites distributed across six orbital planes with a 55° inclination. This configuration allows for global coverage by ensuring that satellites are evenly spread out around the Earth.
- High Altitude: Each NAVSTAR satellite orbits over 20,000 km above Earth, nearly three times the planet's radius. This high altitude positioning contributes to the satellites' ability to cover vast areas efficiently and accurately.
- Redundancy and Reliability: To mitigate the risk of satellite failures impacting coverage, spares are launched to maintain a 24-satellite constellation without interruption. Additionally, the satellites are designed to outlast their anticipated operational lifetimes, enhancing the overall reliability of the network.
The meticulous design of satellite orbits and coverage not only facilitates global positioning capabilities but also ensures continuous and dependable service for various applications. This optimized arrangement within the space segment demonstrates how the segment includes and actually performs to achieve seamless connectivity and precision in satellite networks.
Satellite Constellations and Networks
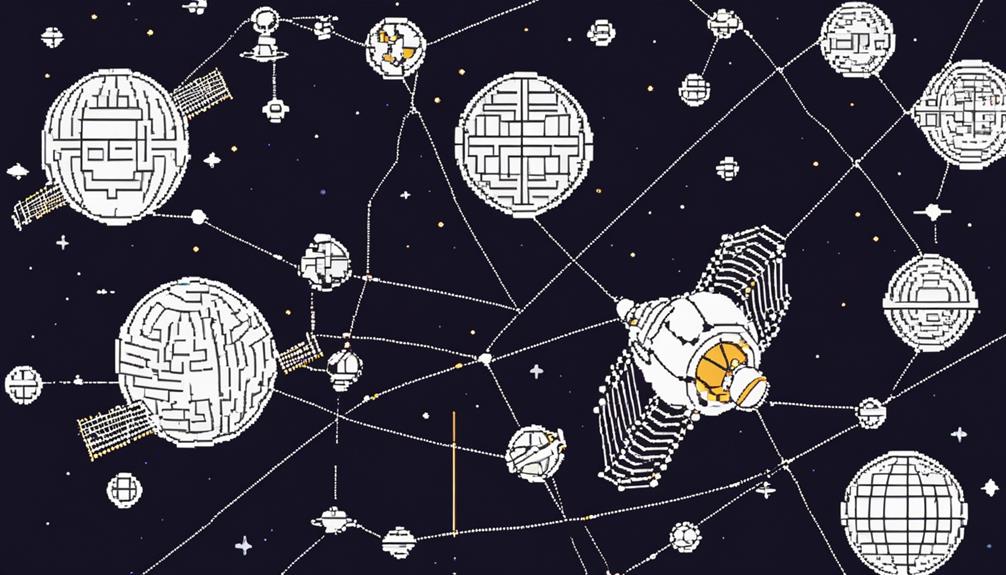
Satellite constellations and networks represent a sophisticated array of interconnected satellites strategically positioned in orbit to provide seamless global coverage and enhance communication reliability across various applications. These constellations, consisting of multiple satellites working together, ensure continuous coverage and redundancy in satellite connectivity. By strategically distributing satellites in orbit, they enable global coverage and communication reliability, making them vital components in the network architecture of satellite systems.
One of the key features of satellite constellations is their ability to facilitate inter-satellite communication and data relay. Satellites within a constellation communicate with each other to efficiently relay signals and data, enhancing the overall performance and resilience of the network. This interconnected communication system enables seamless connectivity for various applications such as telecommunications, navigation, and Earth observation.
On-Orbit Satellite Operations
On-orbit satellite operations encompass critical tasks such as satellite maneuvering techniques, solar panel deployment, and payload activation sequences. These operations are essential for managing satellites effectively in space and ensuring optimal performance for communication and navigation purposes. Coordinating these activities meticulously is crucial for maintaining satellite positions, monitoring health, and delivering reliable services to users on Earth.
Satellite Maneuvering Techniques
Utilizing advanced propulsion systems, satellite maneuvering techniques play a pivotal role in the precise adjustment and maintenance of orbital trajectories for optimal operational efficiency in satellite networks. The maneuver optimization is crucial for achieving orbital efficiency and ensuring the overall success of satellite missions. Key aspects of satellite maneuvering techniques include:
- Propulsion Systems: Different types of propulsion systems such as chemical thrusters, ion thrusters, and cold gas thrusters are employed to facilitate precise orbital adjustments.
- Maneuver Planning: Careful planning is essential to consider factors like fuel consumption, orbital dynamics, and mission objectives to execute maneuvers effectively.
- Precision Maneuvers: Accurate station-keeping, orbit transfers, and constellation formations require precise control and execution of maneuvers to maintain the desired satellite positions.
Solar Panel Deployment
Solar panel deployment is a critical operation in ensuring the continuous power supply for satellite operations in space. Solar panels are essential for power generation capabilities onboard satellites. Efficiency in solar panel design is crucial to maximize power output. Deployment mechanisms must be meticulously planned to ensure proper positioning and functioning of the solar panels. Solar cells are often arranged in series-parallel configurations to optimize energy production. The deployment of solar panels is a key aspect of on-orbit satellite operations, providing the necessary energy for the satellite's electronic systems. Proper solar panel deployment is vital for the functionality and longevity of satellites in space, highlighting the significance of this operation in satellite missions.
Payload Activation Sequence
The initialization of the satellite's payload systems post-orbit involves a meticulously planned and executed sequence known as the payload activation process. This process ensures the proper integration of the payload with the satellite bus systems and is critical for enabling the satellite to perform its intended functions in space. The payload activation sequence consists of:
- Payload Testing and Integration Process: Sequential checks and tests are conducted to verify the functionality of the payload components and their seamless integration with the satellite systems.
- Activation Verification and Functional Checks: Powering up the payload components to verify their functionality and performance to ensure they meet the mission requirements.
- Operational Readiness and Space Functionality: The final step involves ensuring that the payload is ready for operational use in space, guaranteeing its space functionality and mission success.
Satellite Launches and Deployments
In satellite networks, the successful placement and deployment of satellites into their designated orbits are crucial steps for ensuring operational functionality. Prior to launch, extensive launch preparations and safety measures are undertaken to guarantee a successful deployment process. Satellite deployment accuracy is paramount, as even minor deviations can have significant implications on the mission's success. Challenges such as orbital drift, collisions with space debris, and communication disruptions must be meticulously considered and mitigated to ensure optimal satellite positioning.
Once deployed, satellites may require post-deployment adjustments to fine-tune their orbits for optimal performance. Orbit optimization techniques are employed to ensure that satellites are positioned correctly relative to each other and to the Earth. These adjustments are critical for maintaining the overall functionality and efficiency of the satellite network. Deployments can involve releasing satellites individually or as part of a constellation, depending on the mission objectives and network requirements. The precision and success of satellite deployments play a pivotal role in the effectiveness of satellite missions and communication networks, highlighting the significance of meticulous planning and execution in the satellite launch and deployment process.
Satellite Payloads and Instruments
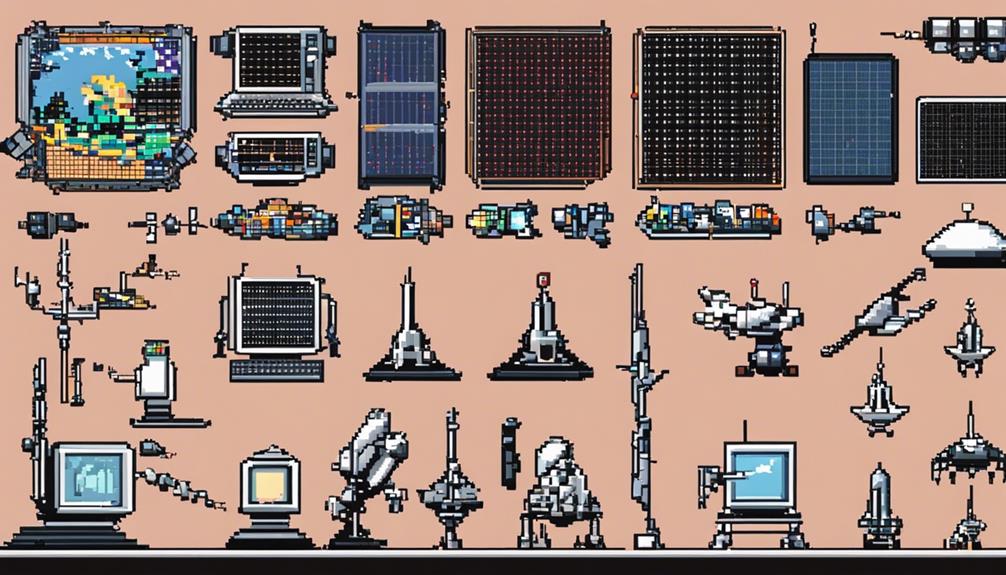
Deployed satellites in satellite networks are equipped with essential components known as satellite payloads and instruments, which serve specific functions critical to the satellite's mission objectives and operational capabilities. These payloads are crucial for various tasks ranging from communication to scientific research. Here are some key points to consider:
- Payload Integration and Testing: Before launch, payloads undergo rigorous integration processes to ensure they work seamlessly together. Testing is vital to verify the functionality and compatibility of each component within the satellite system.
- Payload Performance and Optimization: Once in orbit, payload performance is optimized to enhance the satellite's overall operational efficiency. Fine-tuning parameters and configurations can lead to improved data transmission, image capturing, or scientific measurements.
- Payload Innovation and Advancements: Continuous innovation in payload technology drives advancements in satellite capabilities. From developing more sensitive sensors for Earth observation to increasing data transmission rates for communication services, ongoing improvements in payloads enhance the satellite's versatility and mission potential.
Efficient payload integration, testing, performance optimization, and innovation are essential for maximizing the utility and success of satellites in fulfilling their designated tasks in space.
Satellite Communication Systems
Facilitating seamless communication between Earth-based stations, satellite communication systems comprise essential components onboard satellites to receive, process, and transmit signals for various applications. These systems play a vital role in enabling global connectivity for telecommunication, broadcasting, and data transmission. Satellite communication systems utilize transponders to receive signals from Earth, process them, and retransmit them back to designated locations. The architecture of these systems includes payload components for specific services like television broadcasting and internet connectivity, as well as bus components for power systems, attitude control, and thermal control to support overall functionality.
Satellite Signal Processing | Spacecraft Communication | Satellite Network Architecture |
---|---|---|
Utilizes transponders onboard satellites to process signals. | Facilitates communication between Earth-based stations and satellites. | Defines the structure and design of satellite communication systems. |
Satellite Telemetry and Control
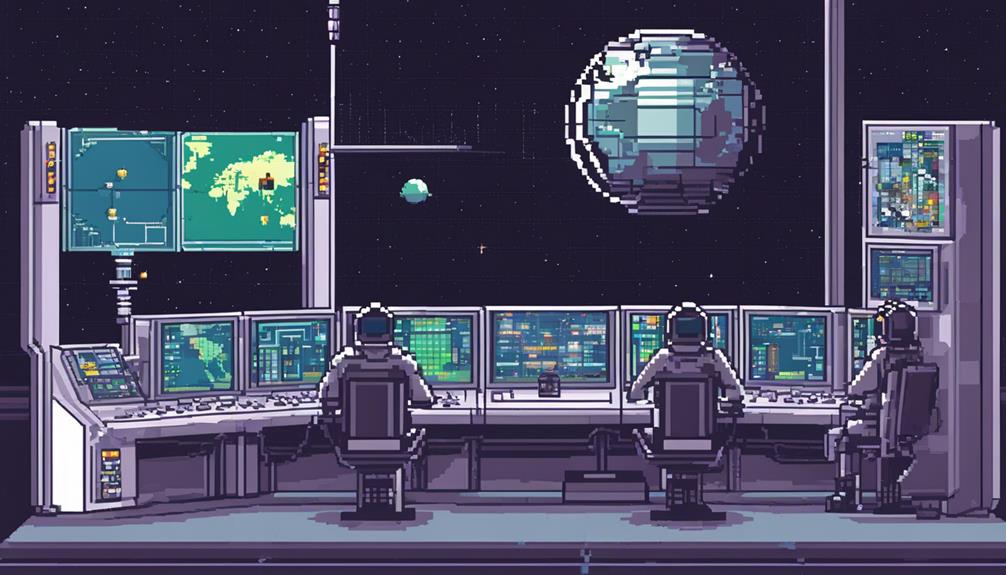
Satellite telemetry and control encompass critical operations in managing satellite functions. Commanding satellite functions and monitoring satellite health are integral components of satellite telemetry and control systems. These activities ensure the proper functioning and maintenance of satellites in orbit.
Commanding Satellite Functions
Monitoring and controlling satellite functions through telemetry and command operations is a critical aspect of satellite network management. When it comes to commanding satellite functions, several key practices come into play:
- Implementing satellite troubleshooting techniques to identify and resolve operational issues promptly.
- Conducting remote satellite operations for tasks like orbit adjustments and payload management from ground stations.
- Focusing on satellite performance optimization through continuous monitoring and adjustment of operational parameters.
These actions are essential for ensuring the smooth operation, efficiency, and longevity of satellites within a network, highlighting the importance of effective telemetry and control procedures in satellite communication systems.
Monitoring Satellite Health
Efficient satellite telemetry and control systems are integral components of ensuring the continuous monitoring and maintenance of satellite health and performance. Satellite telemetry involves the collection of data related to the satellite's health, status, and performance, including parameters such as temperature, voltage levels, and system status. Control systems play a crucial role in managing satellite functions like orbit control, power distribution, and payload operations. Ground stations receive telemetry data for real-time monitoring and analysis, enabling adjustments to satellite operations based on feedback to ensure optimal performance. Key aspects monitored include battery efficiency to maintain power levels, antenna alignment for proper signal reception, and addressing signal interference to uphold communication quality. Monitoring satellite health through telemetry and control systems is essential for the overall functionality and longevity of satellites in orbit.
Satellite Maintenance and Upgrades
Ensuring the continuous operation and longevity of the satellite network involves proactive measures such as launching spares as primary satellites age to prevent interruptions in the 24 satellite constellation. Satellite maintenance and upgrades are crucial aspects of satellite network management to guarantee optimal performance. Key strategies in this domain include:
- Satellite Refueling and Upgrades: Regular maintenance operations may involve refueling satellites to extend their operational lifespans. Additionally, upgrading satellite components such as sensors or transponders can enhance the overall capabilities of the satellite network.
- Extended Satellite Lifespans: Satellites are constructed with robust designs, allowing them to surpass their expected lifespans. By implementing maintenance routines and upgrades, satellite operators can ensure that these satellites continue to function efficiently for extended periods.
- Satellite Constellation Optimization: Continual evaluation of the satellite constellation's configuration is essential for maximizing coverage and performance. Adjusting satellite positions or introducing new satellites into the network can optimize the overall functionality of the constellation.
These maintenance and upgrade practices not only contribute to the reliability and sustainability of satellite networks but also pave the way for future advancements in satellite technology. By prioritizing these efforts, satellite operators can uphold the operational integrity of the network and meet the evolving demands of users worldwide.
Satellite End-of-Life Disposal
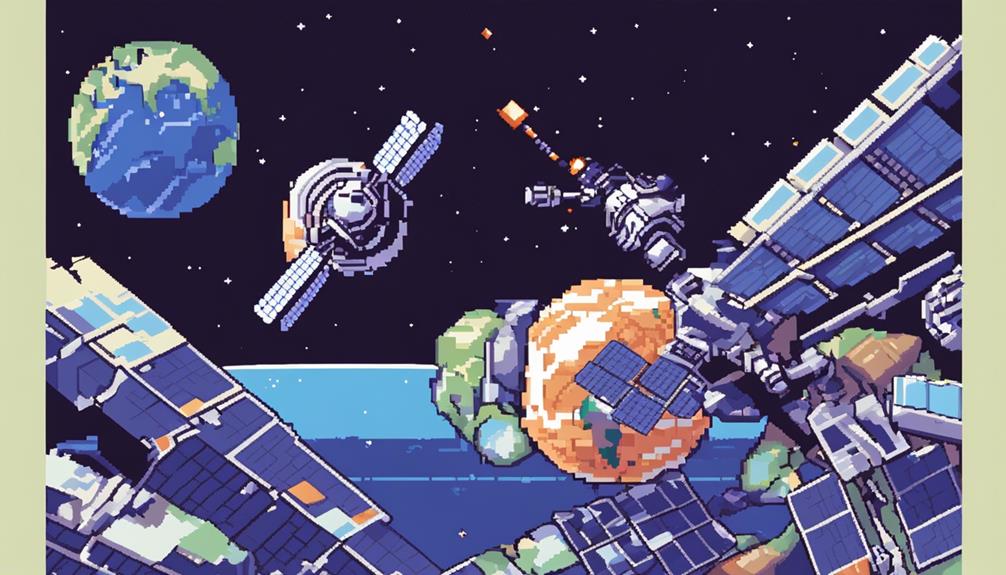
Implementing proper satellite end-of-life disposal procedures is crucial in minimizing space debris and ensuring responsible environmental practices in the satellite industry. Sustainable disposal methods involve deorbiting satellites at the end of their operational life to burn up upon reentry into the Earth's atmosphere. This process significantly reduces the amount of debris in orbit, thereby mitigating the risk of collisions with active satellites or other space objects.
Satellites are carefully maneuvered into controlled reentry trajectories to facilitate safe disposal. By complying with international guidelines and regulations, satellite operators can ensure that the end-of-life disposal process is conducted responsibly. Responsible satellite disposal practices aim to limit the environmental impact of defunct satellites, promoting sustainability in space activities.
The adoption of proper disposal methods not only safeguards operational satellites but also contributes to the long-term sustainability of space operations. By prioritizing environmental impact mitigation and adhering to established protocols, the satellite industry can actively work towards reducing space debris and preserving the orbital environment for future generations. It is imperative for satellite operators to prioritize responsible satellite disposal to uphold the integrity and sustainability of satellite networks.
Future Trends in Satellite Technology
The evolution of satellite technology is prominently marked by the emergence of smaller, more efficient satellites known as 'smallsats' or CubeSats. These advancements in satellite technology are shaping the future of space exploration and communication. The following trends are driving the innovation in satellite technology:
- Satellite Propulsion Advancements: The development of more advanced propulsion systems is revolutionizing satellite maneuverability in space. These systems enable satellites to navigate more effectively, enhancing orbital operations and extending satellite longevity.
- AI in Satellite Technology: The integration of artificial intelligence (AI) and machine learning algorithms is enhancing satellite capabilities in data processing, analysis, and decision-making. AI enables satellites to autonomously optimize their operations, leading to more efficient utilization of resources and improved overall performance.
- Lightweight Satellite Components: Innovations in materials science and manufacturing techniques are leading to the production of lighter, more durable satellite components. These lightweight materials reduce the overall weight of satellites, resulting in decreased launch costs and increased mission flexibility.
These trends collectively represent the future landscape of satellite technology, where efficiency, intelligence, and adaptability are at the forefront of innovation. By harnessing these advancements, the next generation of satellites will meet the increasing demands for higher data transmission rates, improved signal processing, and enhanced cybersecurity measures in satellite networks.
Frequently Asked Questions
What Is the Space Segment of a Satellite?
The space segment of a satellite refers to the physical components positioned in orbit around the Earth. This includes the spacecraft itself, along with various subsystems such as power, communications, thermal control, and attitude control. Additionally, the satellite may carry multiple payloads to cater to diverse applications and customers. Understanding the fundamentals of satellite communication, orbital dynamics, and antenna technology is essential for effective operation within the space segment.
What Are the Segments of the Space System?
When considering the segments of the space system, one must delve into the intricate realm of orbital dynamics, communication links, satellite coverage, frequency allocation, transponders, and earth stations. These components form the backbone of satellite operations, ensuring seamless connectivity and data transmission across vast distances. Understanding the interplay of these segments is crucial for optimizing satellite network performance and achieving efficient data delivery in the ever-evolving landscape of space technology.
What Are the Three Segments of Satellite?
Satellites are categorized into three main segments: Satellite orbits determine their path around the Earth, influencing coverage areas. Satellite coverage refers to the geographic regions where the satellite signals can reach. The satellite link budget involves calculating and optimizing the signal strength to ensure effective communication between the satellite and ground stations. These segments are vital for the seamless operation and performance of satellite networks.
What Is the Space Segment of GPS Satellite Navigation?
The space segment of GPS satellite navigation comprises a constellation of 24 operational satellites in six orbital planes, each orbiting at an altitude exceeding 20,000 km above Earth. This configuration ensures global coverage with a 55° inclination and precise positioning accuracy. The orbital period of these satellites is 12 sidereal hours, maintaining a 4-minute difference from Mean Solar Time. The inclusion of orbital spares guarantees uninterrupted operation of the 24 satellite network.